Top Applications of Restriction Enzymes in modern science
Restriction enzymes, also known as molecular scissors, have revolutionized modern science by enabling precise DNA manipulation. These enzymes, derived from bacteria, cut DNA at specific sequences, paving the way for groundbreaking discoveries and innovations in molecular biology, biotechnology, and medicine. The discovery of restriction enzymes marked a turning point in molecular biology. In the 1960s, scientists Werner Arber, Hamilton O. Smith, and Daniel Nathans uncovered how certain bacteria use these enzymes as a defense mechanism against viral infections. Restriction enzymes recognize and cut DNA at specific sequences, known as restriction sites, preventing the propagation of viruses in bacterial cells. This groundbreaking discovery earned the trio the Nobel Prize in Medicine in 1978 and opened doors to modern genetic engineering. Restriction enzymes operate by binding to specific DNA sequences and cleaving the phosphodiester bonds within the DNA backbone. Their precision is unparalleled, as they can differentiate between foreign DNA and the host organism's DNA. This ability stems from methylation, a protective modification present in host DNA but absent in foreign DNA. Scientists categorize restriction enzymes into different types based on their recognition sequences, cleavage patterns, and requirements for cofactors. Restriction enzymes are classified into three main types: Type I, Type II, and Type III, each with unique properties. These enzymes recognize specific DNA sequences but cut the DNA at random sites far from the recognition sequence. They require ATP and other cofactors, making their mechanism complex and less commonly used in research. Type II enzymes are the most commonly used in laboratories. They cleave DNA at defined positions within or near their recognition sequences. Their simplicity and precision make them invaluable for applications like cloning, gene editing, and sequencing. These enzymes recognize specific sequences and cut DNA at a defined distance from the recognition site. They also require ATP, though their functionality is less versatile compared to Type II enzymes. Restriction enzymes are essential tools for DNA mapping, enabling scientists to cut DNA into manageable fragments for analysis. Gene cloning, a cornerstone of genetic research, relies on restriction enzymes to insert target genes into plasmids, creating recombinant DNA. In polymerase chain reaction (PCR) workflows, restriction enzymes assist in ensuring target specificity by processing DNA for amplification. In genetic engineering, they facilitate the creation of transgenic organisms by precisely altering genetic material. Recombinant DNA technology depends on restriction enzymes to insert foreign genes into vectors like plasmids. This technique is the foundation for producing insulin, growth hormones, and other biopharmaceuticals. The development of GMOs, such as pest-resistant crops, is made possible by restriction enzymes. These enzymes help integrate beneficial traits into an organism’s genome, addressing challenges like food scarcity and agricultural sustainability. In medical research, restriction enzymes are critical for designing vectors that deliver therapeutic genes to patients. Personalized medicine, which tailors treatments to an individual's genetic makeup, leverages these tools to advance precision healthcare. Restriction enzymes play a pivotal role in forensic science by generating unique DNA fragment patterns, which are used in DNA fingerprinting to identify individuals in criminal investigations. The production of vaccines, monoclonal antibodies, and therapeutic proteins relies on restriction enzymes for manipulating DNA. These advances have revolutionized medicine and improved patient outcomes. Molecular diagnostics often employ restriction enzymes to detect genetic mutations associated with diseases like cystic fibrosis and sickle cell anemia, enabling early diagnosis and intervention. Synthetic biology harnesses restriction enzymes to assemble genetic circuits and engineer microorganisms with customized functions, driving innovations in energy, healthcare, and agriculture. While not a traditional restriction enzyme, CRISPR-associated proteins like Cas9 have been inspired by the principles of DNA recognition and cleavage. CRISPR technology has revolutionized genome editing with its unparalleled precision and efficiency. Advances in bioinformatics have enabled researchers to design synthetic restriction enzymes with enhanced functionality. These tools are accelerating discoveries in genomics and proteomics. Restriction enzymes are being explored for environmental remediation efforts, such as engineering bacteria to degrade pollutants and clean up oil spills. The use of restriction enzymes in creating GMOs raises concerns about ecological balance and unintended consequences. Rigorous safety assessments are essential to mitigate risks. Gene editing technologies enabled by restriction enzymes pose ethical dilemmas, particularly in human germline editing. Balancing innovation with ethical responsibility remains a pressing challenge. Restriction enzymes facilitate DNA mapping and the creation of recombinant DNA, which is crucial for producing therapeutic proteins and vaccines. They help integrate beneficial traits into crops and other organisms to address food security and sustainability. Restriction enzymes play a role in developing personalized medicine and gene therapy techniques for treating genetic disorders. DNA fingerprinting relies on these enzymes to generate unique DNA patterns for identification. Restriction enzymes are used to engineer microorganisms and produce advanced medical treatments. Restriction enzymes continue to transform modern science, with their applications expanding across disciplines. Future advancements in synthetic biology and computational biology promise to unlock even greater potential, addressing global challenges in health, environment, and sustainability. FAQs on Top Applications of Restriction Enzymes Restriction enzymes are used in gene cloning, DNA mapping, forensic science, and the production of genetically modified organisms. They cut DNA at specific sequences, allowing scientists to insert or modify genetic material with precision. Type II restriction enzymes are proteins that recognize specific DNA sequences (usually palindromic) and cut at or near these sites. They do not require ATP for activity and are widely used in molecular biology for DNA cloning and analysis. Examples include EcoRI and HindIII. Yes, they are used in molecular diagnostics to identify genetic mutations linked to various disorders. Ethical issues include potential misuse in human gene editing and ecological risks associated with GMOs. 6.What are the different types of regulatory enzymes? Regulatory enzymes control metabolic pathways by responding to various signals, ensuring homeostasis. They include allosteric enzymes, which change activity when effectors bind to their allosteric sites, and covalently modified enzymes, regulated by chemical modifications like phosphorylation. Zymogens are inactive precursors activated by proteolytic cleavage, while isoenzymes are enzyme variants with distinct properties adapted for specific tissues. Additionally, feedback-inhibited enzymes are controlled by the pathway's end product, and some rely on regulatory subunits or molecules like cAMP for activation. These mechanisms allow precise and dynamic regulation of cellular processes.History and Discovery of Restriction Enzymes
How Restriction Enzymes Work
Classification of Restriction Enzymes
Type I Restriction Enzymes
Type II Restriction Enzymes
Type III Restriction Enzymes
Role of Restriction Enzymes in Molecular Biology
DNA Mapping and Gene Cloning
Restriction Enzymes in PCR and Genetic Engineering
Applications in Genetic Research and Biotechnology
Production of Recombinant DNA
Creating Genetically Modified Organisms (GMOs)
Gene Therapy and Personalized Medicine
Forensic Science and DNA Fingerprinting
Industrial and Medical Applications
Development of Biopharmaceuticals
Diagnosing Genetic Disorders
Synthetic Biology
Emerging Innovations Using Restriction Enzymes
CRISPR Technology: A Game Changer
Bioinformatics and Computational Biology
Environmental Applications: Bioremediation
Ethical Implications and Challenges
Risks of Genetic Modification
Ethical Concerns in Biotechnology
Key applications include:
1. Gene Cloning and Recombinant DNA Technology:
2. Genetically Modified Organisms (GMOs):
3. Gene Therapy and Medicine:
4. Forensic Science:
5. Synthetic Biology and Biopharmaceuticals:
Conclusion and Future Prospects
1. What are restriction enzymes used for?
2. How do restriction enzymes aid in genetic engineering?
3. What are Type 2 restriction enzymes?
4. Can restriction enzymes diagnose diseases?
5. What are the ethical concerns with using restriction enzymes?
Recent Posts
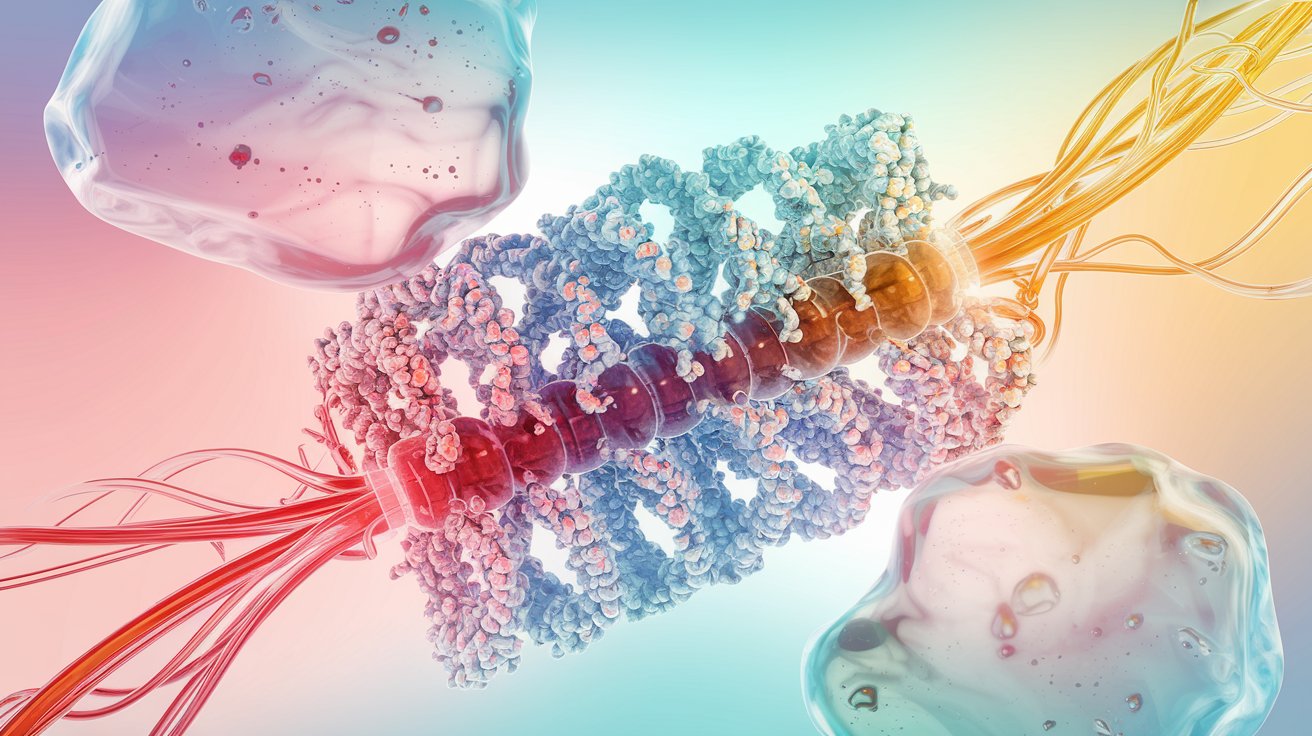
LTCC is one type of calcium channels with long-lasting current during depolarization.LTCC inhibitors are popular and effective clinical drugs in treating cardiovascular diseases. LTCCs are distributed in the brain and involved in neuronal functions such as transmitter release, firing regulation, and brain processing such as reward and memory.
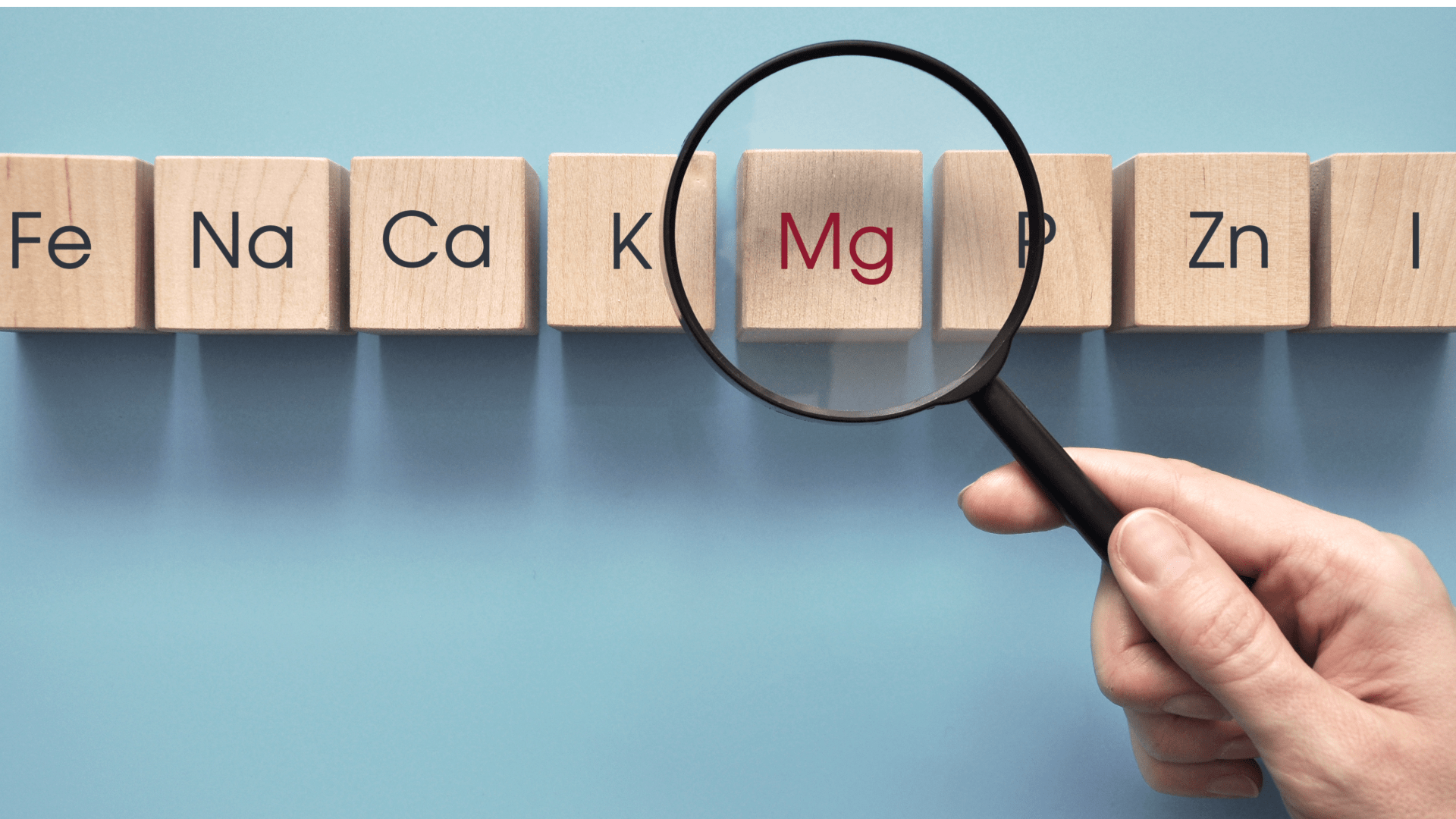
Magnesium acetate is a salt composed of magnesium and acetic acid. It appears as a white, crystalline substance that is highly soluble in water. This compound is used in industrial, medical, and environmental applications due to its stability and reactivity.
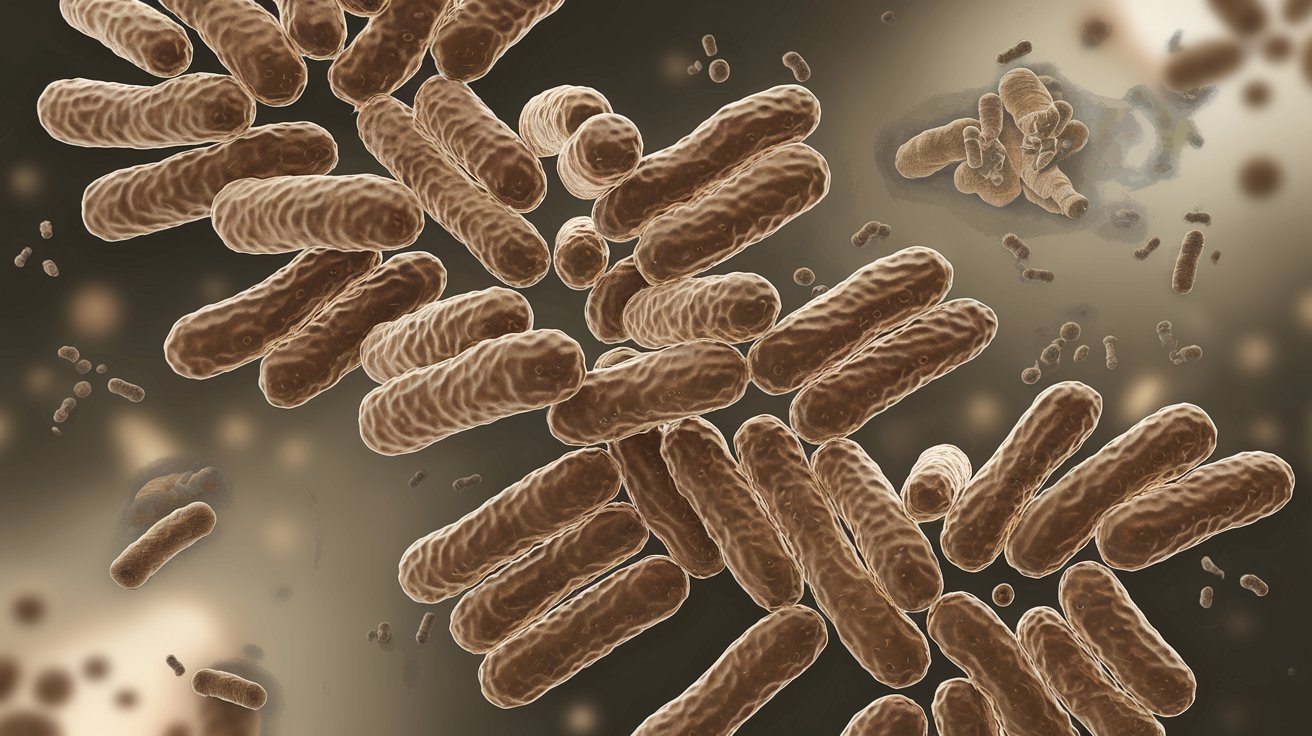
Bacillus amyloliquefaciens, a Gram-positive microbe, has received considerable interest because of its adaptability and extensive uses in industrial, agricultural, medical, and environmental domains. This microorganism is well-known for its capacity to generate enzymes, antimicrobial substances, and other bioproducts, establishing it as a significant resource across multiple areas. The purpose of this review is to examine the various applications of B. amyloliquefaciens, backed by recent research findings.
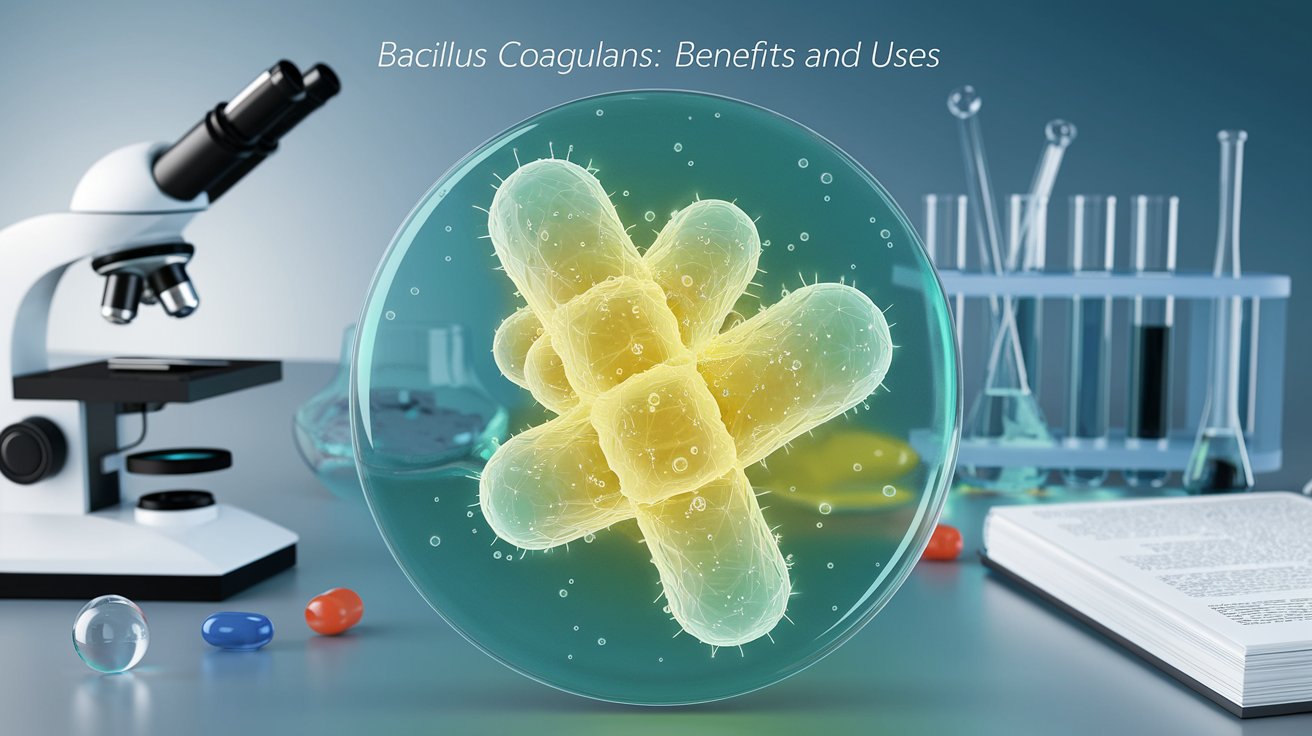
Bacillus coagulans has frequently been reported as an effective treatment for irritable bowel syndrome (IBS) and is known for its ability to produce various enzymes that facilitate digestion and excretion. Additionally, it can regulate host symbiotic microbiota and inhibit the growth of pathogenic bacteria, contributing to its probiotic benefits.
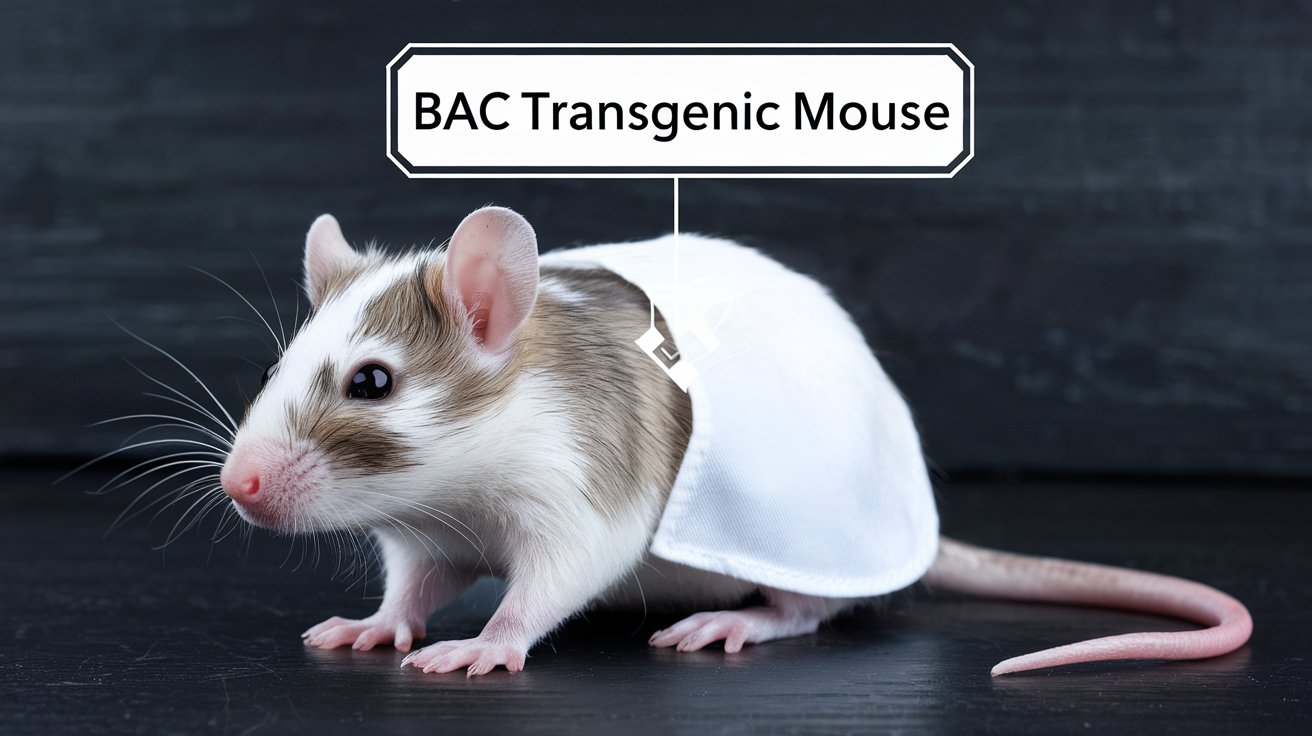
Bacterial Artificial Chromosome (BAC) transgenic mice play a crucial role in the study of genes and diseases, providing benefits that surpass conventional transgenic techniques. BACs enable the inclusion of substantial DNA fragments, encompassing complete genes along with their regulatory components, thereby promoting accurate gene expression in targeted tissues. This technology has proven essential across multiple disciplines, such as neuroscience and immunology, and has contributed to the creation of models that replicate human illnesses.