Exploring the Functionality of L-Type Calcium Channels
In 1985, Yale researchers discovered L-type calcium channels (LTCCs), crucial for physiological processes like excitation-contraction coupling in skeletal muscle. Known as Cav1 Ca2+ channels, they were the first voltage-gated calcium channels to be purified, consisting of five subunits, with the α1 subunit forming the pore and binding to regulatory compounds, particularly dihydropyridine (DHP) drugs. The LTCC family includes four isoforms Cav1.1, Cav1.2, Cav1.3, and Cav1.4 each differing in composition and tissue distribution, with Cav1.1 in skeletal muscle and Cav1.4 in the retina, while Cav1.2 and Cav1.3 are found in the heart and brain. Cav1.2 has a higher activation threshold and greater sensitivity to DHPs compared to Cav1.3, which activates at lower voltages. These isoforms play distinct roles in neuronal signaling, with Cav1.3 aiding in spontaneous firing and both isoforms contributing to burst firing in dopamine neurons. Various LTCC antagonists, including DHPs and other compounds, have been developed for therapeutic applications. L-type calcium channels are complex proteins that play a crucial role in various cellular functions. L-type calcium channels are composed of multiple subunits, primarily the pore-forming alpha (α) subunits and accessory subunits. This heteromultimeric structure is essential for their functionality in the cardiovascular system and other tissues. The α subunits are responsible for forming the channel's pore, allowing calcium ions to flow into the cell. These subunits interact with accessory subunits to create functional calcium channels. The accessory subunits, such as the β and α2δ subunits, play significant roles in modulating the channel's properties. They are involved in the regulation of channel activity and stability, influencing how the channel responds to various signals. The function of L-type calcium channels is regulated by receptor-mediated signal transduction events. These events often lead to the phosphorylation of the channel proteins, which can alter their activity. However, the biochemical details of these regulatory mechanisms are still not fully understood. Studies on chimeric and mutant channels have identified specific regions within the α subunit that are critical for ion selectivity, activation, and inactivation. This highlights the intricate design of the channel that allows it to perform its functions effectively. The α2 and δ peptides are derived from a single gene product, with the α2 peptide being highly glycosylated and hydrophilic. This glycosylation is important for the channel's function, and the structure suggests a receptor-like role for the α2 subunit. Defects in the structure or function of L-type calcium channels can lead to various diseases, emphasizing their importance in maintaining cellular calcium homeostasis and overall health. L-type calcium channels are complex structures composed of multiple subunits that work together to regulate calcium influx in response to cellular signals, with significant implications for health and disease. L-type calcium channels play a crucial role in muscle contraction, particularly in cardiac, skeletal, and smooth muscle cells. Here are the key points regarding their function: L-type calcium channels are the main pathway for calcium ions (Ca²⁺) to enter muscle cells. This influx of calcium is essential for initiating the contraction process in these muscles. When an action potential reaches the muscle cell membrane, it causes the L-type calcium channels to open. The resulting influx of Ca²⁺ ions triggers a series of events leading to muscle contraction. In cardiac and skeletal muscles, this process is vital for the contraction mechanism. In addition to muscle cells, L-type channels are also found in neurons, particularly on cell bodies and proximal dendrites. They are important for linking electrical excitability to calcium-dependent gene transcription, which can influence muscle function indirectly through neural control. The L-type calcium channel is a multisubunit complex consisting of five distinct polypeptides: α1, α2, δ, β, and γ. The α1 subunit forms the channel itself, which is responsible for voltage sensing and gating, as well as the calcium-selective pore. The α1 subunit undergoes extensive alternative splicing, leading to various isoforms that can affect the biophysical properties of the channels. This splicing can influence how effectively the channels function in muscle contraction and may have implications for muscle-related pathologies. Alterations in the function of L-type calcium channels can lead to various muscle dysfunctions and cardiovascular diseases. Understanding their role in muscle contraction is essential for developing treatments for these conditions. L-type calcium channels are essential for proper cardiac function, playing a pivotal role in the excitation-contraction coupling process in heart muscle cells. L-type calcium channels are the primary route for calcium ions (Ca²⁺) to enter cardiac myocytes (heart muscle cells). When the cardiac action potential occurs, these channels open, allowing Ca²⁺ to flow into the cells. This influx is crucial for initiating muscle contraction, as it triggers the release of additional calcium from the sarcoplasmic reticulum, a process known as calcium-induced calcium release (CICR). L-type calcium channels require significant depolarization to activate, typically opening at membrane potentials above -30 mV. This voltage dependence ensures that the channels are activated during the action potential, aligning calcium entry with the contraction phase of the cardiac cycle. The activity of L-type calcium channels is vital for maintaining the rhythmic contractions of the heart. They contribute to the plateau phase of the cardiac action potential, which is essential for preventing tetany (sustained contraction) and allowing the heart to fill with blood between beats. Dysfunction of LTCCs is linked to conditions like Timothy Syndrome, epilepsy, and Parkinson’s disease, highlighting their clinical significance. Timothy syndrome (TS) is a rare and severe inherited condition characterized by cardiac and extracardiac complications, classified as a variant of long QT syndrome (LQT8). TS is associated with mutations in the CACNA1C gene, leading to a complex phenotype that includes structural heart disease, central nervous system issues, and metabolic irregularities, resulting in a high mortality risk. While comprehensive treatment data is limited, β-blockers are commonly used based on their effectiveness in long QT syndrome, alongside other medications like mexiletine and ranolazine to manage arrhythmias. The implantation of a cardioverter defibrillator is often necessary to prevent sudden cardiac death, especially in young patients. Additionally, careful monitoring for infections and blood glucose levels is essential, as altered immune responses and hypoglycemia pose significant risks. Overall, TS remains a challenging condition with ongoing research needed to enhance understanding and treatment. Pharmacological regulation of calcium channels, particularly L-type calcium channels, is crucial for managing various physiological processes and treating cardiovascular diseases. These are a class of medications that inhibit the activity of L-type calcium channels. They are commonly used to treat hypertension, angina, and certain arrhythmias. By blocking these channels, CCBs reduce calcium influx into cardiac and smooth muscle cells, leading to decreased contractility and vasodilation, which lowers blood pressure and reduces cardiac workload . There are several subclasses of calcium channel blockers, including: These primarily affect vascular smooth muscle, leading to vasodilation. Examples include amlodipine and nifedipine. Such as verapamil, which have a more significant effect on the heart, reducing heart rate and contractility. Like diltiazem, which have intermediate effects on both vascular smooth muscle and cardiac tissue . CCBs bind to specific sites on the L-type calcium channels, stabilizing them in a closed state. This prevents the normal influx of calcium during depolarization, which is essential for muscle contraction and neurotransmitter release. The reduction in intracellular calcium levels leads to decreased muscle contraction and relaxation of blood vessels . The pharmacological regulation of calcium channels is vital in treating conditions such as: Hypertension: By lowering vascular resistance and reducing cardiac output. Angina Pectoris: By decreasing myocardial oxygen demand through reduced heart rate and contractility. Arrhythmias: By stabilizing cardiac electrical activity and preventing abnormal rhythms. L-type calcium channels (LTCCs) play a significant role in various physiological processes, and their modulation has important therapeutic applications. L-type calcium channels are crucial in cardiac and vascular smooth muscle function. Calcium influx through these channels is essential for muscle contraction and relaxation. Calcium channel blockers (CCBs), which inhibit LTCC activity, are widely used to treat hypertension and angina. By reducing calcium entry, these drugs lower blood pressure and decrease myocardial oxygen demand, improving heart function and reducing the risk of cardiovascular events. In neurons, L-type calcium channels are involved in neurotransmitter release and neuronal excitability. Their modulation can influence various neurological conditions. Drugs targeting LTCCs may help manage conditions like epilepsy and chronic pain by altering calcium signaling pathways that contribute to neuronal excitability and synaptic transmission. L-type calcium channels are essential for excitation-contraction coupling in striated muscle. Their dysfunction can lead to muscle disorders such as hypokalemic periodic paralysis and malignant hyperthermia susceptibility. Therapeutic strategies that target LTCCs may help manage symptoms or prevent crises in patients with these muscle disorders. LTCCs also play a role in hormone secretion from endocrine cells. For instance, in pancreatic beta cells, calcium influx through L-type channels is critical for insulin release. Modulating LTCC activity can therefore influence metabolic conditions, including diabetes, by affecting insulin secretion. Certain L-type calcium channel antagonists have been explored for their potential in treating chronic pain conditions. By blocking calcium entry, these drugs can reduce pain signaling pathways and provide relief for patients suffering from neuropathic pain L-type calcium channels have diverse therapeutic applications, particularly in cardiovascular, neurological, and muscle disorders, as well as in hormonal regulation and pain management. Their modulation through pharmacological agents offers significant benefits in treating various pathophysiological conditions. L-Type Calcium Channels are integral to cellular physiology, affecting muscle function, neural signaling, and cardiovascular health. Ongoing research aims to refine therapeutic applications, offering hope for managing numerous medical conditions effectively. The primary function of L-type calcium channels (LTCCs) is to mediate the influx of calcium ions (Ca²⁺) into cells, which is crucial for various physiological processes. In muscle cells, LTCCs facilitate excitation-contraction coupling by allowing Ca²⁺ entry that triggers muscle contraction through the release of calcium from the sarcoplasmic reticulum. In neurons, they play a vital role in neurotransmitter release, linking electrical activity to synaptic communication. Additionally, LTCCs are involved in regulating gene expression and hormone secretion, such as insulin release in pancreatic beta cells, highlighting their importance in both excitable and non-excitable cells. Calcium channel blockers (CCBs) primarily affect L-type calcium channels by inhibiting their activity, which reduces the influx of calcium ions (Ca²⁺) into cells. These blockers, such as dihydropyridines, phenylalkylamines, and benzothiazepines, bind to allosteric sites on the L-type Ca v subunits, leading to a decrease in channel opening and subsequent calcium entry. This action is particularly significant in the cardiovascular system, where CCBs help lower blood pressure and reduce cardiac workload by preventing excessive calcium influx into cardiac and vascular smooth muscle cells. Dysfunction can lead to neurological, cardiovascular, and muscular disorders such as arrhythmias and hypertension. Yes, apart from L-Type, there are T-Type, N-Type, P/Q-Type, and R-Type calcium channels, each with unique physiological roles. Yes, specific LTCC modulators are being explored for chronic pain management, particularly neuropathic pain.1. Introduction to L-Type Calcium Channels
2. Structure and Composition
Heteromultimeric Composition:
Pore-Forming Alpha Subunits:
Accessory Subunits:
Regulatory Mechanisms:
Ion Selectivity and Activation:
Glycosylation and Structure:
Clinical Relevance:
3.Role in Muscle Contraction
Primary Route for Calcium Entry:
Triggering Muscle Contraction:
Location in Neurons:
Complex Structure:
Alternative Splicing Effects:
Pathophysiological Implications:
4.Cardiac Function of L-Type Calcium Channels
Calcium Entry and Contraction:
Voltage Dependence:
Role in Heart Rhythm:
5.Clinical Disorders and L-Type Calcium Channels
Timothy Syndrome
6.Pharmacological Regulation of Calcium Channels
Calcium Channel Blockers (CCBs):
Types of CCBs:
Dihydropyridines:
Phenylalkylamines:
Benzothiazepines:
Mechanism of Action:
Clinical Implications:
7.Therapeutic Applications of L-Type Calcium Channels
Cardiovascular Diseases:
Neurological Disorders:
Muscle Disorders:
Hormonal Regulation:
Pain Management:
8.Conclusion
9.FAQs
1. What is the primary function of L-Type Calcium Channels?
2. How do calcium channel blockers affect L-Type Calcium Channels?
3. What happens when L-Type Calcium Channels malfunction?
4. Are there different types of calcium channels?
5. Can L-Type Calcium Channels be targeted for pain relief?
Recent Posts
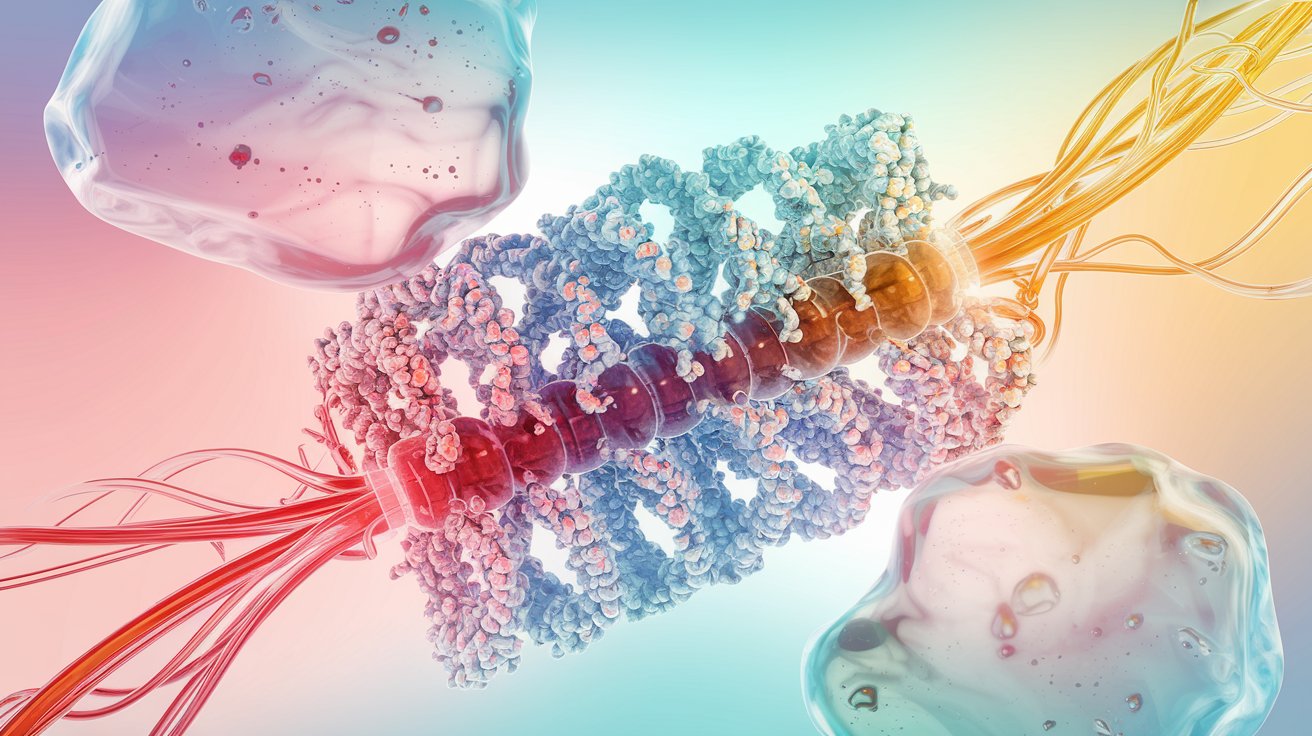
LTCC is one type of calcium channels with long-lasting current during depolarization.LTCC inhibitors are popular and effective clinical drugs in treating cardiovascular diseases. LTCCs are distributed in the brain and involved in neuronal functions such as transmitter release, firing regulation, and brain processing such as reward and memory.
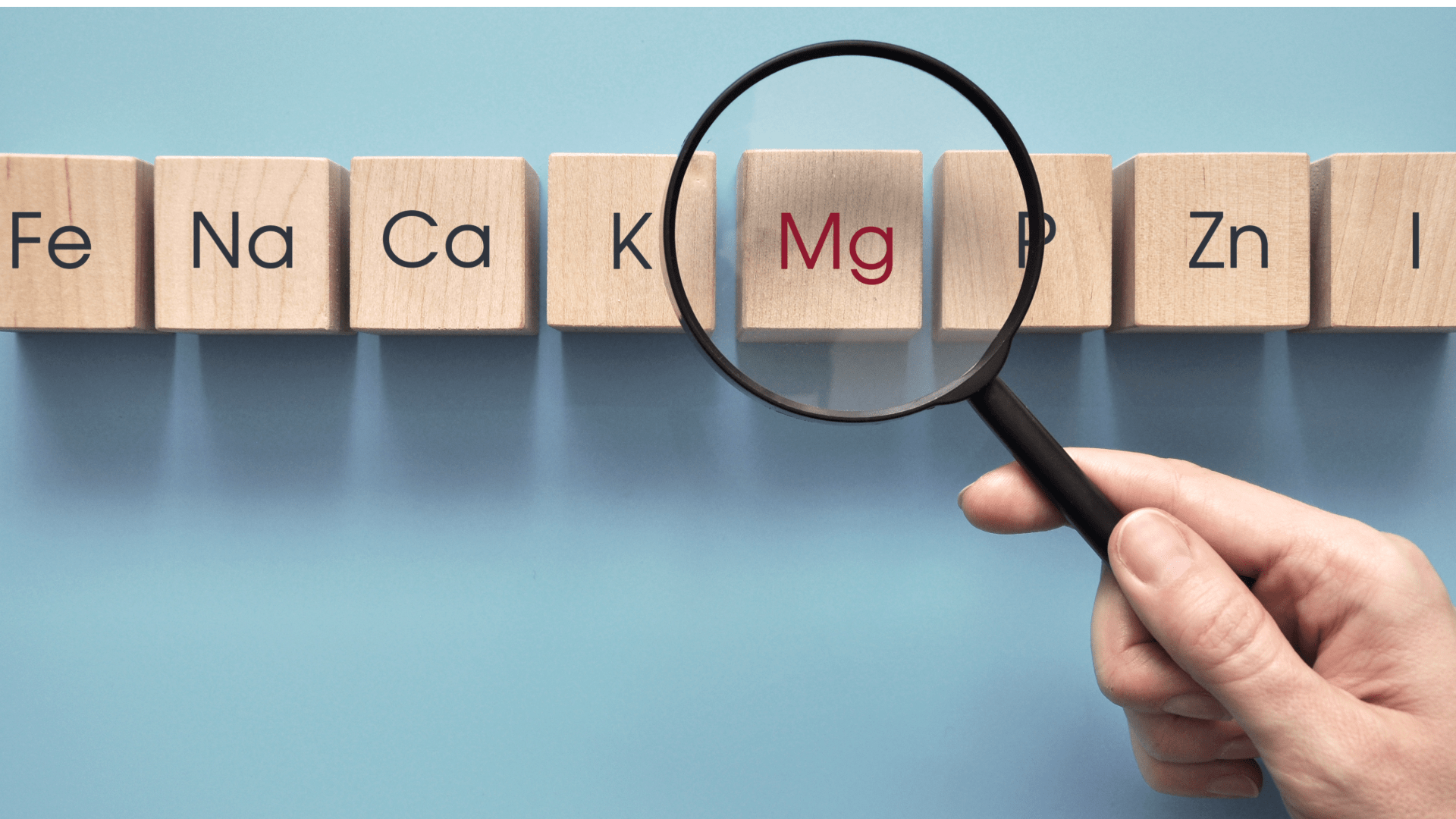
Magnesium acetate is a salt composed of magnesium and acetic acid. It appears as a white, crystalline substance that is highly soluble in water. This compound is used in industrial, medical, and environmental applications due to its stability and reactivity.
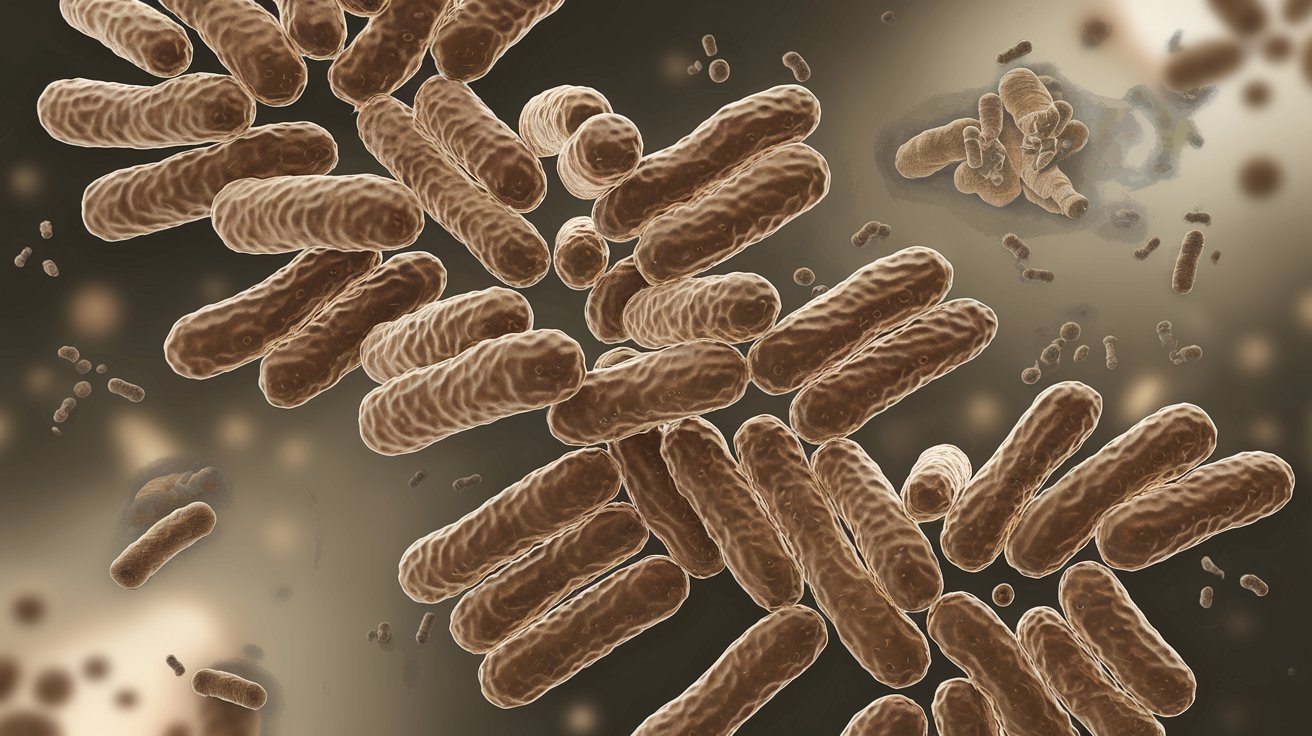
Bacillus amyloliquefaciens, a Gram-positive microbe, has received considerable interest because of its adaptability and extensive uses in industrial, agricultural, medical, and environmental domains. This microorganism is well-known for its capacity to generate enzymes, antimicrobial substances, and other bioproducts, establishing it as a significant resource across multiple areas. The purpose of this review is to examine the various applications of B. amyloliquefaciens, backed by recent research findings.
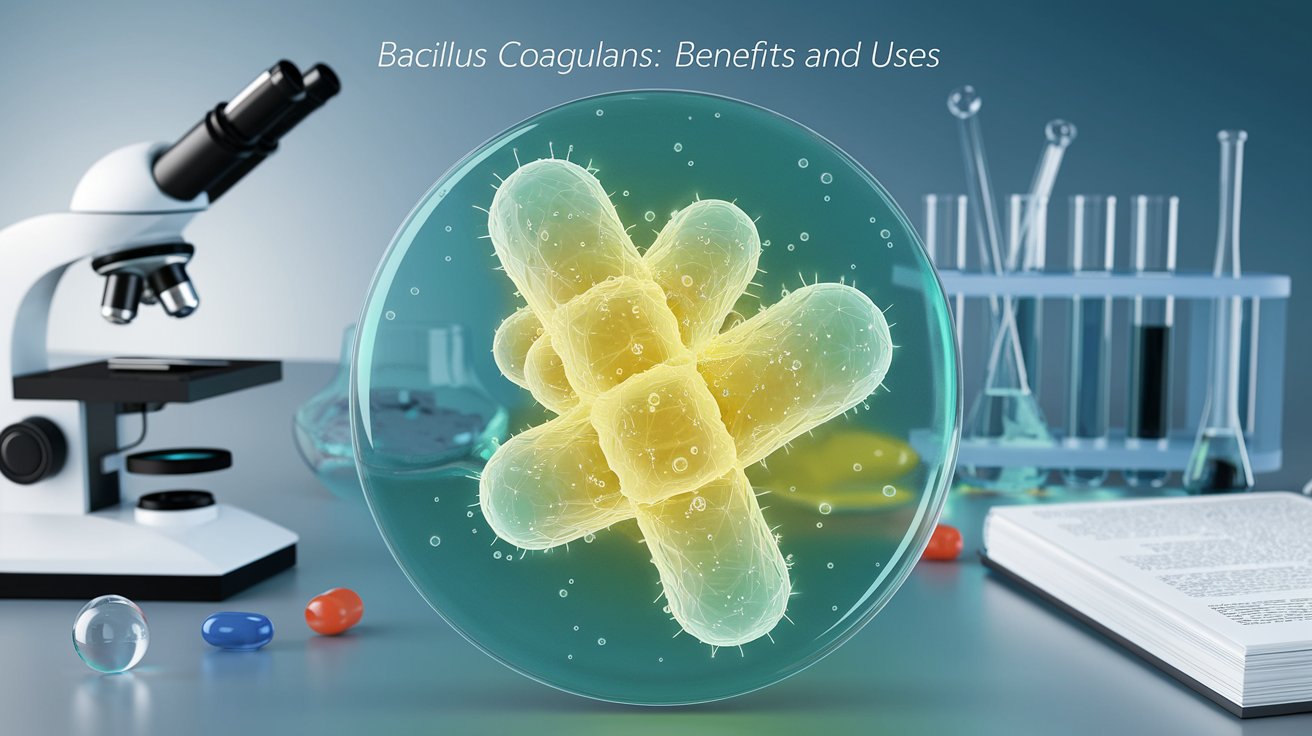
Bacillus coagulans has frequently been reported as an effective treatment for irritable bowel syndrome (IBS) and is known for its ability to produce various enzymes that facilitate digestion and excretion. Additionally, it can regulate host symbiotic microbiota and inhibit the growth of pathogenic bacteria, contributing to its probiotic benefits.
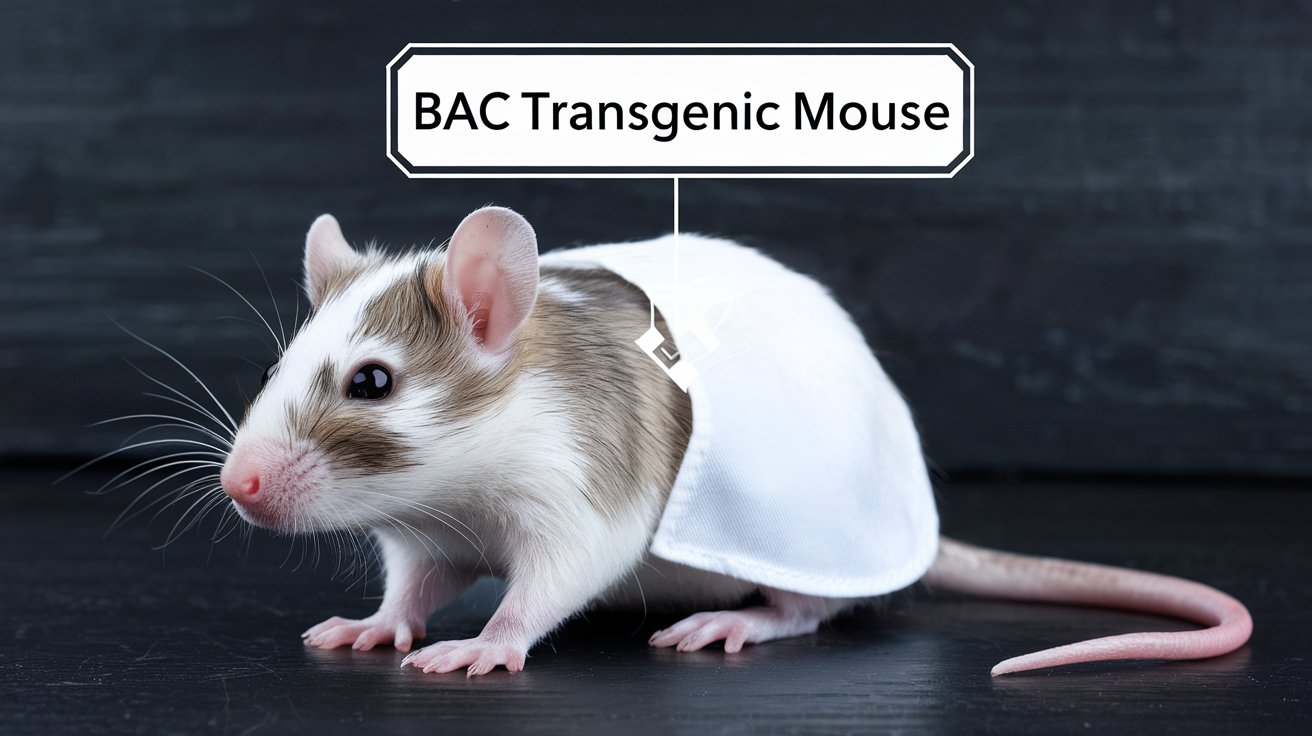
Bacterial Artificial Chromosome (BAC) transgenic mice play a crucial role in the study of genes and diseases, providing benefits that surpass conventional transgenic techniques. BACs enable the inclusion of substantial DNA fragments, encompassing complete genes along with their regulatory components, thereby promoting accurate gene expression in targeted tissues. This technology has proven essential across multiple disciplines, such as neuroscience and immunology, and has contributed to the creation of models that replicate human illnesses.