Acetoacetyl-CoA Essential Intermediate Metabolic Pathways
Acetoacetyl-CoA is a crucial metabolic intermediate involved in multiple biochemical pathways, including fatty acid metabolism, ketogenesis, and cholesterol synthesis. It serves as a key link between lipid and carbohydrate metabolism, playing a vital role in cellular energy production and homeostasis. Acetoacetyl-CoA is a key intermediate in various metabolic pathways, particularly in ketogenesis and ketolysis. It is formed by the condensation of two molecules of acetyl-CoA, a reaction catalyzed by mitochondrial acetyl-CoA acetyltransferase (ACAT1). ACAT1, also known as acetoacetyl-CoA thiolase, plays a crucial role in both the synthesis and breakdown of acetoacetyl-CoA. It can catalyze the reverse reaction, breaking down acetoacetyl-CoA back into two acetyl-CoA molecules, depending on the metabolic needs of the tissue. The direction of the reaction involving acetoacetyl-CoA is influenced by the specific metabolic pathway and tissue type. For instance, during hepatic ketogenesis, ACAT1 favors the formation of acetoacetyl-CoA, while in non-hepatic tissues, it often promotes the breakdown of acetoacetyl-CoA. In addition to its involvement in ketone body metabolism, ACAT1 is also essential in the degradation of isoleucine, where it facilitates the conversion of 2-methylacetoacetyl-CoA into propionyl-CoA and acetyl-CoA. Deficiency in ACAT1 activity leads to an inherited metabolic disorder characterized by ketoacidosis and other clinical symptoms. This condition is associated with mutations in the ACAT1 gene, which can be inherited in an autosomal recessive manner. Recent studies have revealed that ACAT1 has functions beyond its classical enzymatic activity. It has been shown to possess acetyltransferase activity, which allows it to acetylate pyruvate dehydrogenase (PDH), thereby influencing cancer cell metabolism and promoting the Warburg effect, a phenomenon where cancer cells preferentially utilize glycolysis for energy production. Given its involvement in cancer metabolism and drug resistance, ACAT1 is being explored as a potential target for anti-cancer therapies, highlighting its importance in both metabolic regulation and cancer biology. In summary, acetoacetyl-CoA is a vital metabolite with significant roles in energy metabolism and disease, particularly in the context of cancer and metabolic disorders. Acetoacetyl-CoA is a thioester compound formed from the condensation of two acetyl-CoA molecules. Its chemical formula is C4H6O CoA, indicating it contains four carbon atoms, six hydrogen atoms, and one coenzyme A (CoA) moiety. The structure of acetoacetyl-CoA includes: 1) A ketone group (C=O) at the second carbon, which is crucial for its reactivity and role in metabolic pathways. 2) A thioester bond between the acyl group (acetoacetyl) and the CoA, which is important for the energy transfer during metabolic reactions. The acetoacetyl group consists of a carbon backbone with a ketone functional group. The CoA portion is a large, complex molecule that includes a pantothenic acid moiety, a cysteamine unit, and an adenosine diphosphate (ADP) component. This structure allows CoA to participate in various biochemical reactions, acting as a carrier for acyl groups. Acetoacetyl-CoA serves as a key intermediate in several metabolic pathways, including: 1) Ketogenesis: It is produced in the liver during the breakdown of fatty acids and is converted into ketone bodies. 2) Ketolysis: In non-hepatic tissues, it can be broken down to release energy. 3) Isoleucine Degradation: It is involved in the conversion of 2-methylacetoacetyl-CoA into propionyl-CoA and acetyl-CoA, highlighting its versatility in metabolism. Understanding the structure of acetoacetyl-CoA is important in the context of metabolic disorders, such as ACAT1 deficiency, where the inability to properly metabolize this compound can lead to serious health issues, including ketoacidosis and other metabolic disturbances . Acetoacetyl-CoA is a vital metabolite with a complex structure that plays significant roles in energy metabolism and is crucial for various biochemical pathways. The conversion of acetoacetyl-CoA to acetyl-CoA is catalyzed by the enzyme mitochondrial acetyl-CoA acetyltransferase (ACAT1). This enzyme facilitates the breakdown of acetoacetyl-CoA into two molecules of acetyl-CoA through a reversible reaction. 1) In the context of ketolysis, ACAT1's activity is crucial as it breaks down acetoacetyl-CoA to release acetyl-CoA, which can then enter the citric acid cycle for energy production. 2) Conversely, during ketogenesis, the reaction can proceed in the opposite direction, where two acetyl-CoA molecules condense to form acetoacetyl-CoA, depending on the tissue and metabolic needs. 1) The ability to convert acetoacetyl-CoA to acetyl-CoA is vital for energy metabolism, particularly during periods of fasting or low carbohydrate intake when ketone bodies are produced as an alternative energy source. 2) This conversion is also significant in the degradation of isoleucine, where ACAT1 plays a role in the reversible conversion of 2-methylacetoacetyl-CoA into propionyl-CoA and acetyl-CoA, further emphasizing its metabolic versatility. 1) Deficiency in ACAT1 can lead to metabolic disorders, as seen in patients with ACAT1 deficiency, who exhibit symptoms related to impaired ketone body metabolism and isoleucine degradation. 2) Understanding this conversion process is essential for developing therapeutic strategies for metabolic disorders associated with ACAT1 dysfunction. The conversion of acetoacetyl-CoA to acetyl-CoA is a critical metabolic reaction facilitated by ACAT1, playing a significant role in energy production and metabolic regulation. Acetoacetyl-CoA thiolase (EC 2.3.1.9) is an enzyme that typically catalyzes the synthesis of acetoacetyl-CoA through the condensation of two molecules of acetyl-CoA. This reaction is a reversible nondecarboxylative condensation, which means it can proceed in both directions depending on the cellular conditions. In contrast, the enzyme NphT7, identified in the study, synthesizes acetoacetyl-CoA by condensing acetyl-CoA with malonyl-CoA. This is a significant distinction because NphT7 does not exhibit thiolysis activity against acetoacetyl-CoA, which means it does not break down acetoacetyl-CoA into its components. The reaction catalyzed by NphT7 is described as energy-favored, making it an ideal candidate for supplying acetoacetyl-CoA in cellular processes. This is particularly important in metabolic pathways where acetoacetyl-CoA serves as a precursor for various biosynthetic processes. Acetoacetyl-CoA is a crucial precursor in the mevalonate pathway, which is essential for the biosynthesis of terpenoids. The study highlights that NphT7's unique mechanism of action allows it to play a vital role in this pathway, potentially enhancing the production of important compounds like carotenoids and biofuels. The ability of NphT7 to synthesize acetoacetyl-CoA efficiently opens up possibilities for its application in biotechnology. For instance, coexpression of the nphT7 gene with other genes involved in the mevalonate pathway has been shown to significantly increase mevalonate production in engineered strains of bacteria. While acetoacetyl-CoA thiolase is a well-known enzyme in metabolic pathways, the discovery of NphT7 as an acetoacetyl-CoA synthase provides new insights into the diversity of enzyme functions within the thiolase superfamily. This could lead to advancements in metabolic engineering and synthetic biology applications. Acetoacetyl-CoA synthetase (AACS) is a crucial enzyme that plays a significant role in the anabolic utilization of ketone bodies (KBs) for de novo lipid synthesis. It catalyzes the reaction that combines acetoacetate (AcAc), a type of ketone body, with CoA to produce acetoacetyl-CoA (AcAc-CoA) in the cytosol. AACS is highly regulated and is influenced by the metabolic needs of various tissues. In many tissues, its activity is linked to cholesterol requirements, while in adipose tissue, it is more closely associated with fatty acid synthesis. This regulation ensures that lipid synthesis is aligned with the body's metabolic state. AACS is found in several lipogenic tissues, including the liver, adipose tissue, lactating mammary gland, skin, intestinal mucosa, adrenal glands, and the developing brain. These tissues can efficiently utilize KBs for lipid synthesis, often preferring them over glucose. The enzyme has a low micromolar Km for acetoacetate, indicating that the availability of acetoacetate is unlikely to limit its activity in the fed state. This suggests that AACS can effectively utilize KBs when they are present. The utilization of KBs for lipid synthesis is considered glucose-sparing, which is particularly important in low-carbohydrate dietary conditions. This metabolic pathway allows for the conversion of fatty acids into KBs in the liver, which are then used to synthesize cholesterol and long-chain fatty acids in both hepatic and non-hepatic tissues. The study of AACS and its role in lipid interconversion is vital for understanding metabolic processes, especially in contexts such as low-carbohydrate diets and energy metabolism. Further research is needed to explore its quantitative contributions in various metabolic situations. AACS is a key enzyme in lipid metabolism, facilitating the use of ketone bodies for lipid synthesis and playing a significant role in energy homeostasis across various tissues. Acetoacetyl-CoA reductase (PhaB) plays a crucial role in the biosynthesis of polyhydroxyalkanoates (PHAs) by reducing acetoacetyl-CoA to (R)-3-hydroxybutyryl-CoA. This enzymatic step has significant implications in various fields: PHA, particularly poly(3-hydroxybutyrate) [P(3HB)], is a biodegradable plastic that serves as a sustainable alternative to conventional plastics. The reduction of acetoacetyl-CoA by PhaB is essential for producing (R)-3-hydroxybutyryl-CoA, which is a monomer precursor for PHA synthesis. This process highlights the potential of using microbial systems for bioplastic production, contributing to environmental sustainability. The pathway involving PhaB is a part of the broader metabolic processes in bacteria like Cupriavidus necator. The enzyme not only facilitates the conversion of acetoacetyl-CoA to (R)-3-hydroxybutyryl-CoA but also integrates into the metabolic network that allows bacteria to utilize various carbon sources for growth and energy. This aspect of microbial metabolism is vital for understanding how microorganisms can be engineered for enhanced biopolymer production. The reduction of acetoacetyl-CoA is linked to the utilization of fatty acids as substrates for PHA biosynthesis. By converting fatty acids into acetoacetyl-CoA, and subsequently into (R)-3-hydroxybutyryl-CoA through the action of PhaB, bacteria can effectively utilize fatty acids as a carbon source. This process not only aids in biopolymer production but also demonstrates the versatility of microbial systems in converting waste materials into valuable bioproducts. The function of acetoacetyl-CoA reductase is pivotal in the production of bioplastics, the metabolic capabilities of microorganisms, and the utilization of fatty acids, showcasing its importance in both environmental and industrial applications. The conversion of acetoacetyl-CoA to 3-hydroxy-3-methylglutaryl-CoA (HMG-CoA) is a significant step in metabolic pathways, particularly in the context of cholesterol biosynthesis and ketogenesis. Here are the key points regarding its importance: 1) HMG-CoA is a precursor in the mevalonate pathway, which is essential for cholesterol synthesis in the liver. This pathway involves the condensation of acetoacetyl-CoA with a third acetyl-CoA molecule to form HMG-CoA, catalyzed by HMG-CoA synthase (HMGCS). 2) The subsequent reduction of HMG-CoA to mevalonate by HMG-CoA reductase (HMGCR) is a critical step in cholesterol biosynthesis, making this conversion vital for maintaining cholesterol levels in the body. 1) During fasting or low-carbohydrate conditions, the body shifts to ketogenesis, where HMG-CoA plays a crucial role. It is converted into acetoacetate, which can be used as an energy source by various tissues, including the brain. 2) The production of HMG-CoA from acetoacetyl-CoA is essential for generating ketone bodies, which serve as an alternative energy source when glucose availability is low. The reactions catalyzed by HMGCS and HMGCR are exergonic, meaning they release energy and make the overall mevalonate biosynthetic reaction thermodynamically favorable. This ensures that the conversion of acetoacetyl-CoA to HMG-CoA is not only crucial but also efficient in terms of energy utilization. The study highlights that the thiolase and HMGCS enzymes form a complex that channels the intermediate acetoacetyl-CoA directly to HMG-CoA without releasing it into the bulk solvent. This efficient transfer is essential for maintaining the flow of metabolites in the pathway. The conversion of acetoacetyl-CoA to HMG-CoA is crucial for both cholesterol biosynthesis and ketogenesis, playing a significant role in energy metabolism and maintaining lipid homeostasis in the body. Acetoacetyl-CoA is an important molecule in various metabolic pathways, and its synthesis can occur through different enzymatic reactions. The paper discusses a unique enzyme, NphT7, which synthesizes acetoacetyl-CoA in a novel way. Here are the key points regarding acetoacetyl-CoA synthesis as described in the study: The enzyme NphT7, found in the mevalonate pathway gene cluster of Streptomyces sp. strain CL190, is identified as an unusual acetoacetyl-CoA synthesizing enzyme. Unlike typical pathways that use two molecules of acetyl-CoA, NphT7 catalyzes the condensation of acetyl-CoA and malonyl-CoA to produce acetoacetyl-CoA and CoA. The synthesis activity of acetoacetyl-CoA was tested under both monosubstrate and bisubstrate conditions. Under monosubstrate conditions, malonyl-CoA concentrations were varied, while under bisubstrate conditions, both acetyl-CoA and malonyl-CoA were present. The study employed a spectrophotometric assay to monitor the formation of a Mg²⁺-acetoacetyl-CoA enolate complex, which allowed for the determination of steady-state kinetic parameters. The reaction was conducted at 30 °C, and the initial velocities were calculated based on the formation of this complex over time. It was noted that NphT7 cannot utilize malonyl-ACP as a substrate, which is a significant distinction from other KAS III enzymes that can use this substrate. This specificity highlights the unique role of NphT7 in acetoacetyl-CoA synthesis. The reactions were carried out in a controlled environment with specific buffer conditions (100 mM Tris-HCl, pH 8.0, 5 mM MgCl₂, and 1 mM dithiothreitol) to ensure optimal enzyme activity. The concentrations of malonyl-CoA were varied to assess their impact on the synthesis activity. The synthesis of acetoacetyl-CoA by NphT7 represents a novel mechanism within the thiolase superfamily, showcasing the enzyme's unique substrate specificity and reaction conditions. This research contributes to our understanding of metabolic pathways involving acetoacetyl-CoA and highlights the potential for discovering new enzymatic functions in microbial systems. The process of beta oxidation of fatty acids involves the breakdown of fatty acids into acetyl-CoA units within the mitochondria. This process includes the activation of fatty acids, their transport into the mitochondria, and the subsequent removal of two carbon atoms as acetyl-CoA. Fatty acid oxidation is an aerobic mitochondrial process where a fatty acid is converted into acetyl-CoA units. In this pathway, fatty acids (FA) move as derivatives of coenzyme A (CoA) utilizing NAD and FAD. The process initiates with the absorption of fatty acids into the cell. Following this, the activation of FA is essential to propel it into the oxidation pathway. The enzyme acyl-CoA synthetase, which serves as the activator, is found on the surface of the outer mitochondrial membrane and the endoplasmic reticulum (ER). It performs the ATP-dependent thioesterification of fatty acids with coenzyme A. Long-chain acyl-CoA is transported into the mitochondria bound to Carnitine, a quaternary ammonium compound. This entry constitutes the rate-limiting step of beta oxidation. Once inside the mitochondria, beta oxidation of fatty acids occurs, during which two carbon atoms are cleaved off in the form of acetyl-CoA from the acyl-CoA at the carboxyl terminal. The bond between the second carbon (beta carbon) and the third carbon (gamma carbon) is broken, hence the term beta oxidation. The regulation of acetoacetyl-CoA pathways in Escherichia coli is closely linked to the metabolism of acetate and acetyl-CoA, particularly during different growth phases and environmental conditions. Here are some key points regarding this regulation: E. coli cells undergo a metabolic switch when transitioning from exponential growth to stationary phase. During exponential growth on sugars, they excrete acetate via the phosphotransacetylase-acetate kinase (Pta-AckA) pathway, which is reversible and allows for ATP generation. As cells enter the stationary phase, they resorb acetate instead of excreting it. This acetate is then activated to acetyl-CoA by the enzyme acetyl-CoA synthetase (Acs). The generated acetyl-CoA is utilized for energy production and biosynthetic processes through the tricarboxylic acid (TCA) cycle and the glyoxylate shunt. The study suggests that neither acetyl-P nor acetyl-CoA serves as a feedback signal for the regulation of acs transcription. This conclusion is based on observations that mutants lacking AckA or both Pta and AckA show similar acs transcription patterns, indicating that these intermediates do not play a regulatory role. The induction of acs is influenced by several factors, including cyclic AMP levels, oxygen availability, and the activity of various regulators such as the cyclic AMP receptor protein (CRP) and the glyoxylate shunt repressor IclR. The activator FadR also plays a role in this regulatory network. Acetyl-CoA plays a critical catabolic role during mixed acid fermentation and aerobic growth on excess glucose or glycolytic intermediates. Under conditions favoring mixed acid fermentation, acetyl-CoA cannot enter the TCA cycle, highlighting its importance in energy metabolism. The regulation of acetoacetyl-CoA pathways in E. coli is a complex interplay of metabolic switches, regulatory factors, and the roles of key intermediates, which together facilitate the efficient use of acetate and acetyl-CoA for energy and biosynthesis. Acetoacetyl-CoA is a crucial intermediate in the synthesis of ketone bodies, which are produced during periods of fasting or low carbohydrate intake. It is formed from acetyl-CoA and is essential for the production of acetoacetate, one of the primary ketone bodies used for energy during starvation or prolonged exercise. The regulation of acetoacetyl-CoA synthesis is significantly influenced by the enzyme acetyl-CoA acetyltransferase. This enzyme catalyzes the conversion of acetyl-CoA to acetoacetyl-CoA. The activity of this enzyme is modulated by the concentration of its substrates and products, particularly CoASH, which acts as a product inhibitor. The initial-rate kinetics of acetyl-CoA acetyltransferase show a hyperbolic response to changes in acetyl-CoA concentrations, with an apparent Km of approximately 0.237 mM. This indicates that the enzyme is sensitive to acetyl-CoA levels, which is critical for maintaining appropriate rates of ketogenesis. CoASH, as a product inhibitor, introduces complex kinetics into the reaction, resulting in four intermediary plateau regions. This means that as CoASH levels increase, the enzyme's response to acetyl-CoA concentrations becomes less sensitive, which can affect the overall rate of acetoacetyl-CoA synthesis and, consequently, ketogenesis. The concentration of acetoacetyl-CoA and its regulation are vital for the liver's ability to respond to increased acetyl-CoA levels, especially when fatty acids are mobilized, such as during fasting or high-fat diets. This regulatory mechanism ensures that ketone body production is appropriately elevated when needed. The study suggests that the control of acetoacetyl-CoA synthesis by acetyl-CoA concentrations, influenced by CoASH, is a key regulatory mechanism in ketogenesis. This control system allows the liver to adaptively manage energy production from ketone bodies based on metabolic demands. Acetoacetyl-CoA plays a pivotal role in ketogenesis, with its synthesis being tightly regulated by enzymatic activity and product inhibition, ensuring that the liver can efficiently produce ketone bodies in response to metabolic needs. Acetyl-CoA is a crucial metabolite that intersects with various metabolic pathways. It serves as a key indicator of the cell's metabolic state, influencing numerous biological regulatory mechanisms through protein acetylation modifications. In a "growth" or "fed" state, high levels of nucleocytosolic acetyl-CoA promote lipid synthesis and histone acetylation, which are essential for cell growth and proliferation. Conversely, during "survival" or "fasted" states, acetyl-CoA is primarily directed into the mitochondria. This shift supports ATP synthesis and the production of ketone bodies, which serve as alternative energy sources when carbohydrates are scarce. Cells can sense acetyl-CoA levels through protein acetylation modifications. The abundance of these modifications reflects the metabolic state of the cell, allowing for the regulation of various protein activities. This sensing mechanism is vital for adapting to changes in nutrient availability. The acetylation of proteins can either inhibit or activate their functions. For instance, the acetylation of certain metabolic enzymes can be responsive to glucose levels, linking them to intracellular acetyl-CoA abundance. Sirtuins, a family of deacetylases, play a critical role in reversing acetylation modifications, thus restoring enzyme activity when acetyl-CoA levels are high. Fluctuations in acetyl-CoA levels not only regulate acetylation modifications but also influence broader cellular functions. For example, during starvation, lower nucleocytosolic acetyl-CoA levels can limit fatty acid synthesis and promote autophagy, a process crucial for cellular survival under stress. Ongoing research aims to uncover the intricate mechanisms by which acetyl-CoA and other acyl-CoA metabolites influence cellular metabolism and regulation. Understanding these processes is essential for elucidating their roles in health and disease. Acetyl-CoA is a pivotal metabolite that not only serves as a substrate for various metabolic processes but also acts as a signaling molecule that helps cells adapt to changing metabolic states. Current research explores acetoacetyl-CoA’s role in: 1. Targeting metabolic diseases, 2. Developing bioengineered compounds, 3. Enhancing biofuel and bioplastic production. Acetoacetyl-CoA is a fundamental molecule in energy metabolism, lipid biosynthesis, and ketogenesis. Its role in human health and disease highlights its importance in biochemical research and medical advancements. Acetoacetyl-CoA is a key metabolic intermediate in fatty acid metabolism, cholesterol synthesis, and ketone body production. It is broken down by thiolase enzymes into two molecules of acetyl-CoA, which enter the citric acid cycle. It is converted to HMG-CoA, a precursor for cholesterol biosynthesis in the liver. During fasting, acetoacetyl-CoA is used to form ketone bodies, an alternative energy source for the brain and muscles. Yes, its imbalance is linked to metabolic disorders like diabetes, obesity, and lipid storage diseases. Key enzymes include thiolase, synthetase, reductase, and HMG-CoA synthase, all of which control its metabolic fate.1.Introduction
Definition and Formation:
Enzymatic Role:
Metabolic Pathways:
Role in Isoleucine Degradation:
Clinical Significance:
Recent Findings:
Potential as a Therapeutic Target:
2.Acetoacetyl-CoA Structure
Chemical Composition:
Functional Groups:
Molecular Structure:
Role in Metabolism:
Clinical Relevance:
3.Acetoacetyl-CoA to Acetyl-CoA
Enzymatic Reaction:
Metabolic Pathways:
Physiological Importance:
Clinical Implications:
4.Understanding Acetoacetyl-CoA Thiolase and NphT7
Function of Acetoacetyl-CoA Thiolase:
Comparison with NphT7:
Energy-Favored Reaction:
Role in the Mevalonate Pathway:
Implications for Biotechnology:
5.Understanding Acetoacetyl-CoA Synthetase (AACS)
Definition and Function:
Regulation:
Tissue Distribution:
Kinetic Properties:
Metabolic Implications:
Research Significance:
6.Implications of Acetoacetyl-CoA Reductase in PHA Biosynthesis
Bioplastic Production:
Microbial Metabolism:
Fatty Acid Utilization:
7.Acetoacetyl-CoA to HMG-CoA
Cholesterol Biosynthesis:
Ketogenesis:
Thermodynamic Favorability:
Enzymatic Coupling:
8.Understanding Acetoacetyl-CoA Synthesis
Enzyme Identification:
Reaction Conditions:
Kinetic Parameters:
Substrate Specificity:
Experimental Setup:
9.Acetoacetyl-CoA in Beta-Oxidation
10.Regulation of Acetoacetyl-CoA Pathways in Escherichia coli
Metabolic Switch:
Resorption of Acetate:
Role of Acetyl-P and Acetyl-CoA:
Regulatory Factors:
Catabolic Role:
11.Regulation and Role of Acetoacetyl-CoA in Ketogenesis
Key Role in Ketogenesis:
Regulatory Mechanism:
Kinetic Properties:
Influence of CoASH:
Physiological Relevance:
Control Mechanism:
12.Acetyl-CoA: A Key Regulator of Metabolism
Role of Acetyl-CoA in Metabolism:
Growth vs. Survival States:
Sensing Acetyl-CoA Levels:
Acetylation and Deacetylation:
Impact on Cellular Functions:
Future Directions:
13.Future Research and Applications
14.Conclusion
15.Frequently Asked Questions (FAQs)
1. What is the function of acetoacetyl-CoA?
2. How is acetoacetyl-CoA converted to acetyl-CoA?
3. What role does acetoacetyl-CoA play in cholesterol synthesis?
4. Why is acetoacetyl-CoA important in ketogenesis?
5. Can dysregulation of acetoacetyl-CoA metabolism cause diseases?
6. What enzymes regulate acetoacetyl-CoA pathways?
Recent Posts
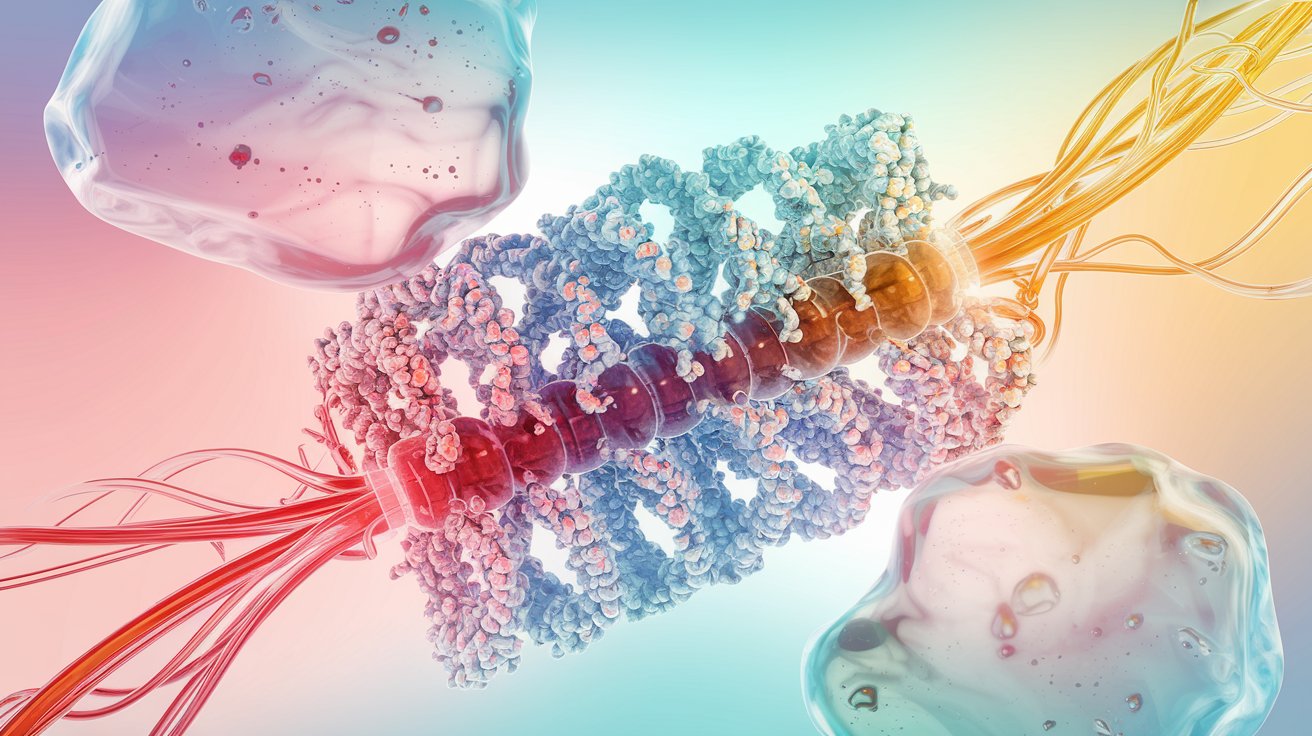
LTCC is one type of calcium channels with long-lasting current during depolarization.LTCC inhibitors are popular and effective clinical drugs in treating cardiovascular diseases. LTCCs are distributed in the brain and involved in neuronal functions such as transmitter release, firing regulation, and brain processing such as reward and memory.
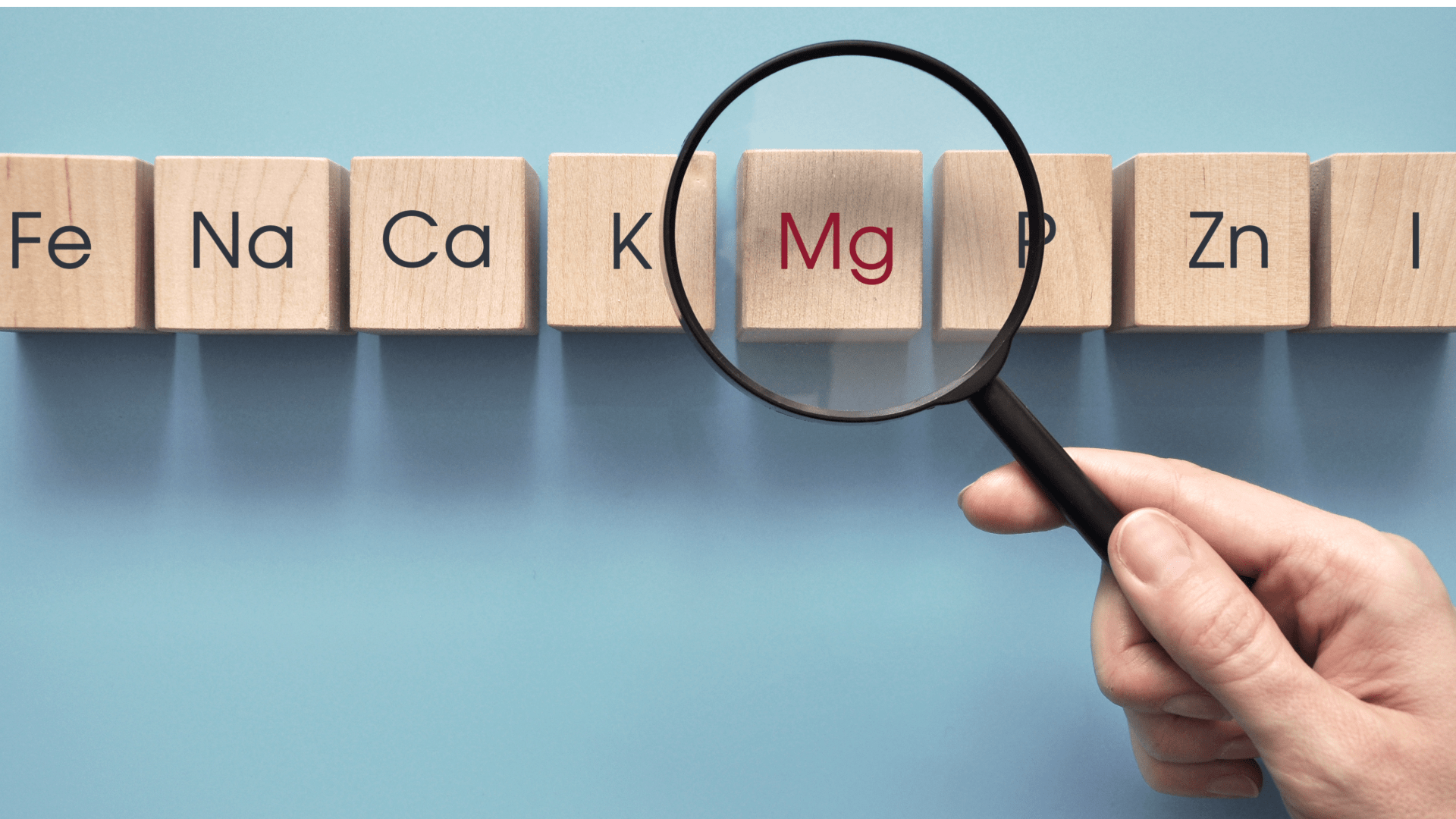
Magnesium acetate is a salt composed of magnesium and acetic acid. It appears as a white, crystalline substance that is highly soluble in water. This compound is used in industrial, medical, and environmental applications due to its stability and reactivity.
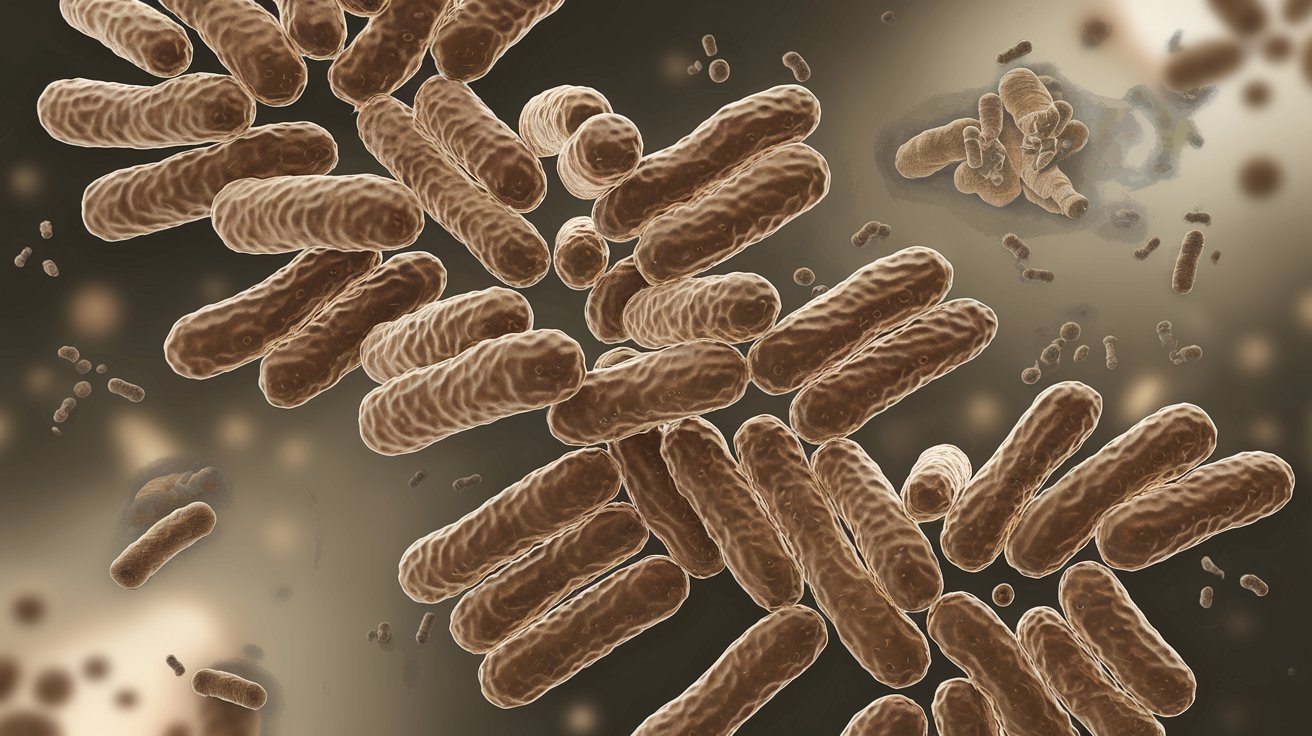
Bacillus amyloliquefaciens, a Gram-positive microbe, has received considerable interest because of its adaptability and extensive uses in industrial, agricultural, medical, and environmental domains. This microorganism is well-known for its capacity to generate enzymes, antimicrobial substances, and other bioproducts, establishing it as a significant resource across multiple areas. The purpose of this review is to examine the various applications of B. amyloliquefaciens, backed by recent research findings.
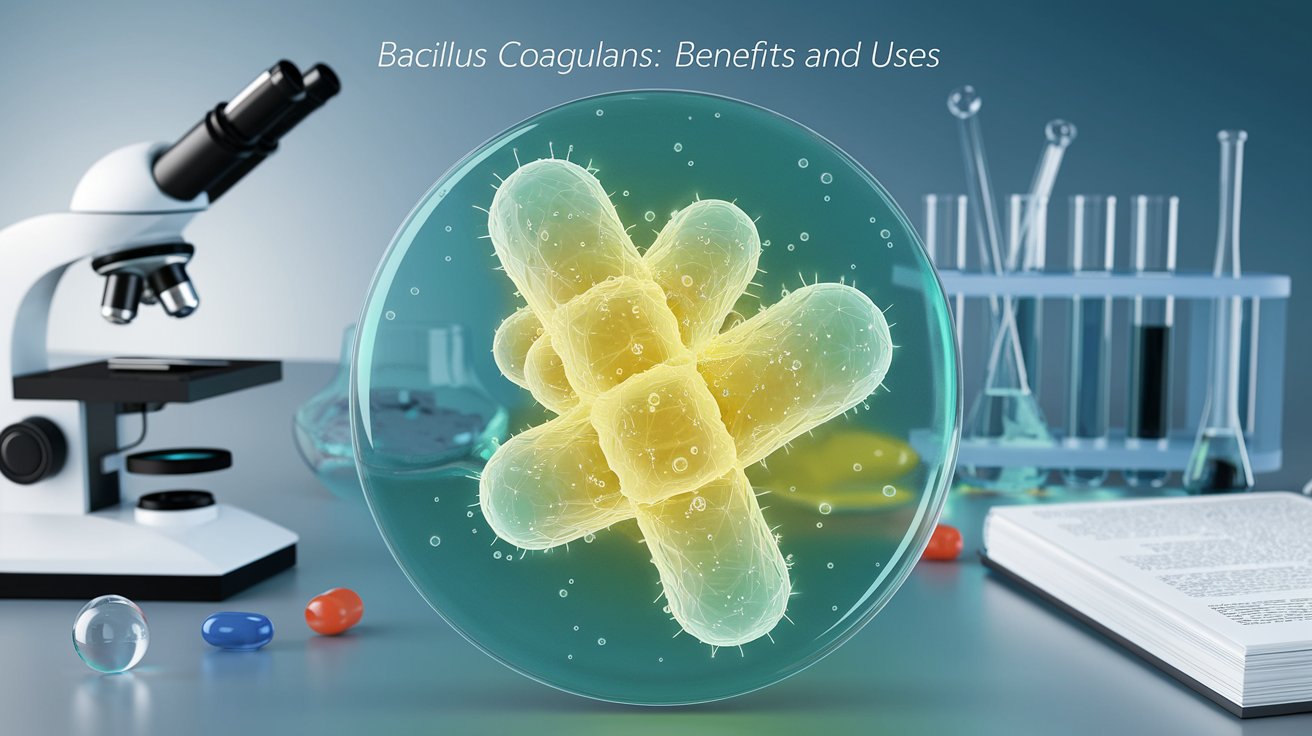
Bacillus coagulans has frequently been reported as an effective treatment for irritable bowel syndrome (IBS) and is known for its ability to produce various enzymes that facilitate digestion and excretion. Additionally, it can regulate host symbiotic microbiota and inhibit the growth of pathogenic bacteria, contributing to its probiotic benefits.
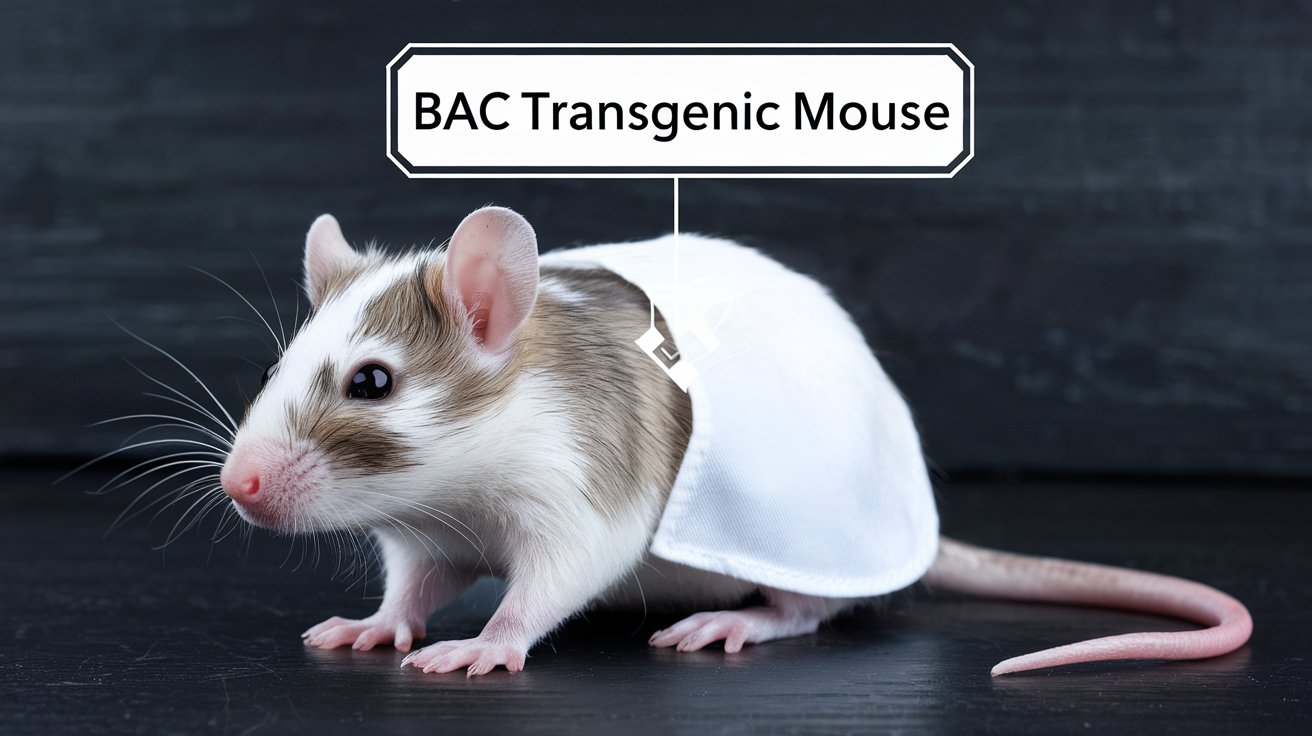
Bacterial Artificial Chromosome (BAC) transgenic mice play a crucial role in the study of genes and diseases, providing benefits that surpass conventional transgenic techniques. BACs enable the inclusion of substantial DNA fragments, encompassing complete genes along with their regulatory components, thereby promoting accurate gene expression in targeted tissues. This technology has proven essential across multiple disciplines, such as neuroscience and immunology, and has contributed to the creation of models that replicate human illnesses.